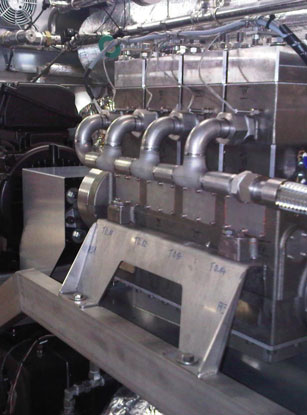
Heat-to-power
At a WWTP, heat is mainly required to heat the digestor which operate at temperatures between 35-38°C, but also for heating of buildings or specific processes such as ammonia stripping. Heat is also produced on-site in CHP plants or gas boilers, which are usually operated with the biogas produced in anaerobic digestion. The overall heat balance of a typical WWTP is often positive, meaning that excess heat is blown into the air because it exceeds the demand on-site, and heat sale to external customers is not feasible.
This excess heat can be partially valorised with processes that turn heat into electricity (“heat-to-power”), drawing on excess or low-grade heat of the WWTP and increasing electricity production at the plant. Available technologies for heat-to-power include steam or organic rankine cycle units, or thermoelectric generators. The economic feasibility of these units also depends on the actual seasonal heat profile of the WWTP with varying amount of excess heat in summer and winter operation.
-
What purposes?
-
Which technologies?
-
Where?
Technologies
Steam Rankine Cycle/Organic Rankine Cycle
When operating a CHP plant at a WWTP, heat is available in the cooling water and exhaust gas. While a large part of this heat is used for internal purpose (e.g. heating of digestor), surplus heat is wasted as excess to the atmosphere via exhaust gas or plate coolers. This heat can be valorised by heat-to-power technologies such as steam rankine cycle (SRC) and organic rankine cycle (ORC).
These technologies convert low-grade heat into electricity using a thermal working fluid as heat carrier, which is steam in SRC and an organic fluid of high molecular weight in ORC. This working fluid is pumped to a boiler where it is evaporated, passed through an expansion device (turbine or other expander) to drive a generator, and then through a condenser heat exchanger where it is finally re-condensed. The choice of the working fluid highly depends on the temperature level and operational characteristics at which the process works to gain maximum efficiency. SRC is suitable to utilise heat at high temperature (e.g. exhaust gas), while ORC can also be adapted to use heat at lower temperature (e.g. cooling cycle). Usually, a SRC or ORC process can convert excess heat to power with an overall efficiency of 12-20% depending on working fluid and size of the system. Economic feasibility of SRC/ORC systems depends on the capital cost of the system and annual operating time, i.e. seasonal availability of excess heat.
- German association of ORC technology
- Orcan: supplier of ORC technology
- Power Generation with the Siemens ORC-Module
Thermoelectric generators
Thermoelectric conversion is a heat-to-power technology under development that can be applied to improve the electrical performance of a cogeneration plant (CHP). The thermoelectric generator (TEG) is integrated into the exhaust line of the CHP engine, replacing the conventional exhaust gas heat exchanger. It partially converts exhaust gas heat into electricity by utilising the physics of the Seebeck effect. Remaining heat energy is recovered like in a conventional heat exchange system.
Due to the absence of any movable parts, the maintenance requirements for TEG are very low. However today, the electricity production is still considerably lower in comparison with other commercially available heat-to-power technologies (e.g. based on Organic-Rankine-Cycle). Once the thermoelectric conversion technology is leveraged by high volume production like e.g. for automotive or consumer markets, a TEG system in a CHP is expected to amortise in about 5 years.